Current efforts to reduce our reliance on fossil fuels, simultaneously curbing atmospheric pollutions and emissions of greenhouse gases in the process, will necessarily lead to an increase in the utilization of renewable energy sources (RES).
These are based on several very different working principles and technologies: while traditional hydropower, “the champion of RES”, is easily adjustable to electricity demand (assuming water is present in the reservoirs), power generation based on solar and wind energy is intrinsically unsteady, alternating periods of over- and undersupply characterized by large-amplitude variations.
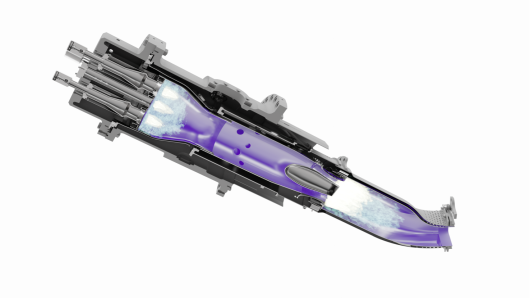
Figure 1 (above) Example of a longitudinally-staged (reheat) combustion concept, the hydrogen-fired Constant Pressure Sequential Combustor (CPSC), implemented in Ansaldo Energia’s GT36 heavy-duty gas turbine.
However, in practical terms, on-demand and large-scale power generation by hydraulic turbines is limited to specific geographical regions and not generally available everywhere. Therefore, the issue of robustly balancing demand and supply of electricity within the energy system will become increasingly challenging as the amount of unsteady RES attached to the electric grid increases, as expected, in the decades ahead.
Transitioning to Carbon-Free Fuels
Today, gas turbines play the crucial role of ensuring the stability of modern, complex energy systems because of their ability to quickly ramp up or down, power generation, on-demand and at large scale. In this balancing act gas turbines can provide grid stability across a wide range of time scales, from shorter (days) to longer (seasonal) ones, but, for this crucial purpose, they presently still burn large amounts of natural gas, stored in a vast network of pipelines and underground caverns, significantly contributing to carbon dioxide emissions.
A transition to gas turbines that can burn carbon-free fuels, such as hydrogen, produced either from water electrolysis in periods of excess RES output or from natural gas jointly with carbon capture and storage, can optimally address the challenge of energy system stability while, at the same time, curbing undesired emissions of atmospheric pollutants (NOx, PM) and greenhouse gases (CO2). In such scenarios, it is stocked hydrogen, and not natural gas, that constitutes the energy-storage chemical vector providing us the electricity we need in time periods with insufficient output from wind and solar sources.
The challenge of clean, stable and efficient hydrogen combustion
Gas turbines represent very mature hi-tech machines that, developed and continuously improved over the last 100 years, have been finely tuned for optimal performance with fossil hydrocarbon-based fuels. A desired fuel switch to hydrogen (H2), due to the physical and combustion properties of this molecule being largely different from those of more conventional hydrocarbons, implies a comprehensive re-design of the gas turbine combustion system and significant research and development efforts are ongoing.
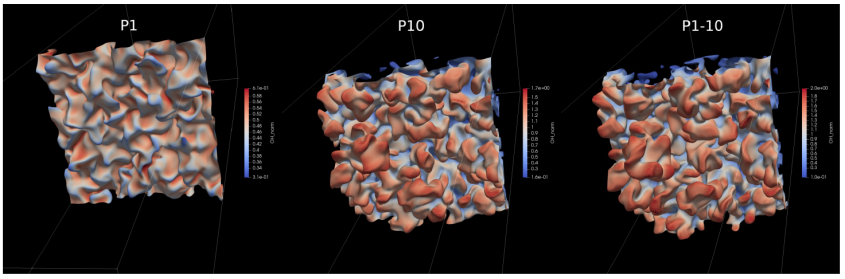
Very expensive testing of the gas turbine combustion system from laboratory-scale to full-size is required in this development. However, despite their large cost, such efforts provide limited fundamental insight into the physics that controls the combustion process at the high pressures present in gas turbines. This is due to obvious difficulties in performing detailed optical measurements and diagnostics inside high-pressure vessels, by design inaccessible devices. Ultimately, the consequences of this situation materialize as costly R&D programs that almost uniquely rely on a trial-and-error procedures based on very limited understanding of the rate-controlling physics.
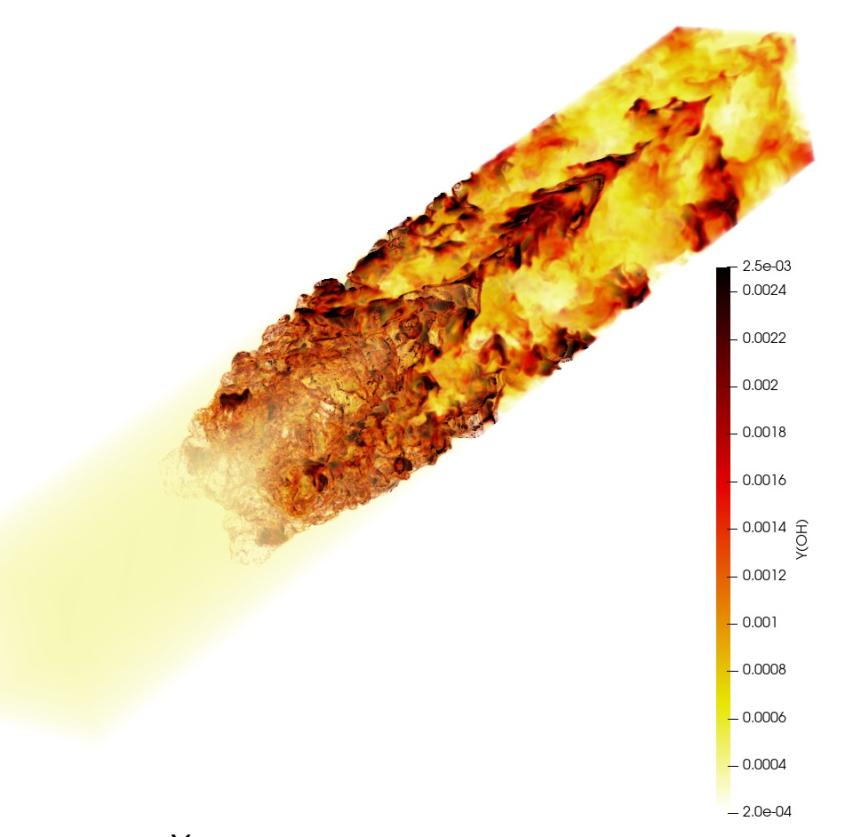
Thanks to recent significant progress in the scale of available High-Performance Computing (HPC) platforms, first-principles numerical simulations of turbulent reactive flows characterized by very high accuracy are becoming possible. These large-scale, high-resolution calculations can potentially revolutionize combustion systems development in industry, proceeding well beyond the peripheral role to which earlier, low-accuracy numerical simulations have been relegated. Of crucial importance in this challenging development endeavours are HPC-enabled simulations that can close existing knowledge gaps in our fundamental understanding of hydrogen premixed combustion, the cleanest approach to efficient power generation in gas turbines.
Massively parallel Direct Numerical Simulations (DNS) of hydrogen flames on supercomputer Betzy
Starting in 2018, the HPC project “HyReheat” (Combustion of hydrogen blends in gas turbines at reheat conditions) has enabled SINTEF researchers to deploy the DNS code ‘’S3D’’, unleashing its full potential on up to 60000 CPU cores of the Betzy supercomputer, and perform first-principles Direct Numerical Simulations (DNS) to accurately model and investigate turbulent premixed hydrogen flames at conditions relevant to gas turbines (high reactants temperature and pressure). DNS calculations accurately resolve all time and length scale of the turbulent reactive flow and, therefore, are considered model-free “numerical experiments” not affected by measurements uncertainty. Accordingly, DNS provides unmatched data completeness (amount of relevant information) about the simulated flame. These novel physical insights, unprecedented in accuracy and resolution, come at a computational cost that, as it turns out, is becoming increasingly affordable.
The sheer size, and number, of the DNS calculations conducted on Betzy within the “HyReheat” project is unprecedented in Norway and on par with the largest efforts in the field of combustion research worldwide (US, Japan and China). The “HyReheat” DNS results, and the fundamental insights they provided, have resulted in 11 highly-cited publications in international peer-reviewed scientific journals, twice as many presentations at international conferences, and, crucially, greatly improved understanding of the intricacies of hydrogen combustion in gas turbines. The present research effort, thanks to Betzy’s computational “muscles”, has already contributed to significant advances in the development of longitudinally-staged (sequential) combustion systems for hydrogen-fired gas turbines and will continue to do so in the years ahead.
[1] Martin Rieth, Andrea Gruber and Jacqueline H. Chen “The effect of pressure on lean premixed hydrogen-air flames”, Combustion and Flame 250 (2023) 112514.